E.ON Energy Research Center Building
The research leading to these results has been partially funded by the European Commission under the European Union’s Seventh Framework Programme (FP/2007-2013)/FP7-ICT-2013.3.4, project LOCAL4GLOBAL under the contract #611538.
Test Case Description:
In order to test and evaluate the decentralized version of PCAO, the new headquarter of E.ON Energy Research Center (E.ON ERC), which is located in Aachen, Germany (see Figure 1) was considered as a testbed. The building is supplied with both renewable and nonrenewable energy sources and has been equipped with modern energy conversion and distribution technologies. The implemented innovative energy concept efficiently meets all different energy demand types of the building subject to satisfying thermal comfort and air quality standards. The energy conversion system of the building is mainly divided into two parts; low-temperature circuit and high-temperature circuit. The heart of the low-temperature circuit is a turbo compressor driven heat pump, which tries to recycle the waste heat of the building.
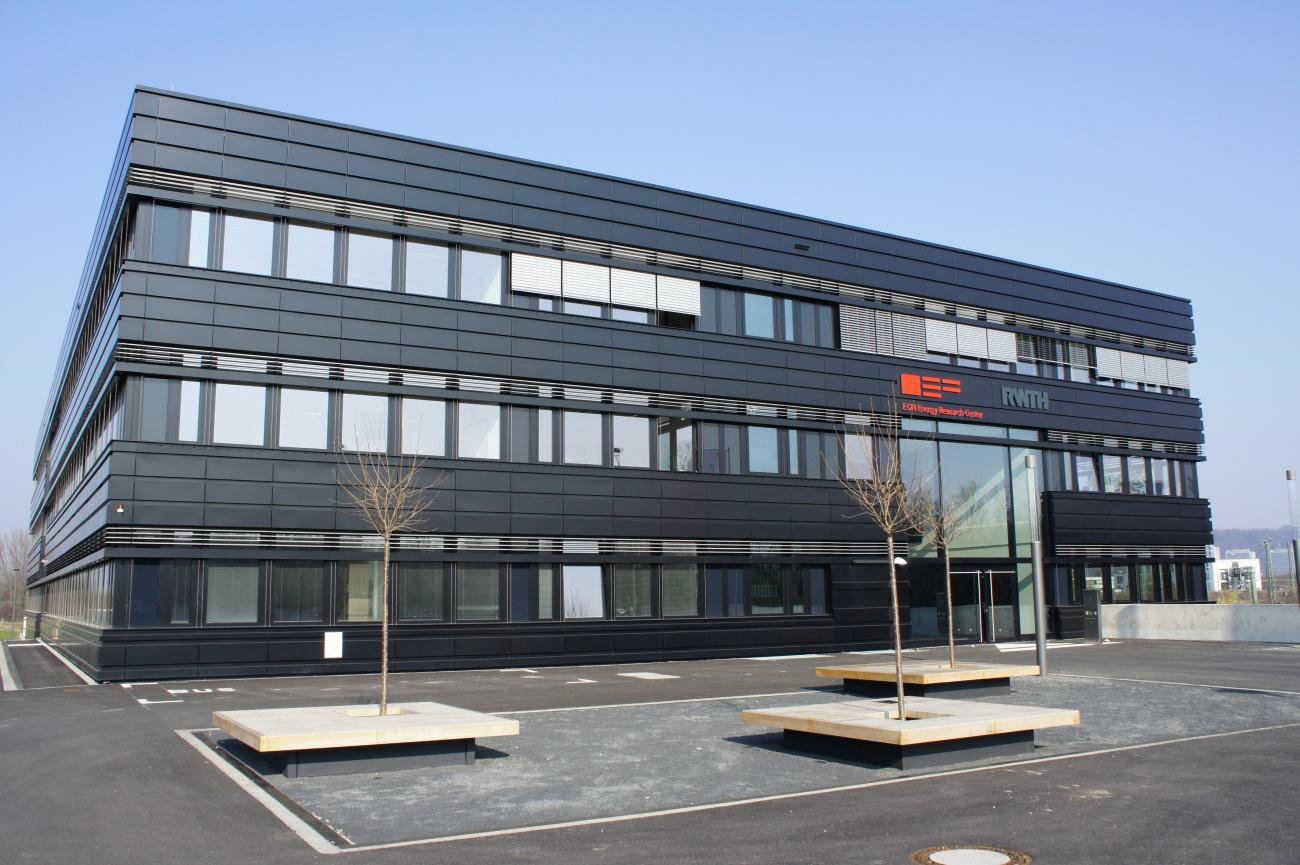
In addition, a glycol cooler and a field of 40 borehole heat exchangers keep the energy balance of the heat pump. The geothermal field serves as an energy source in winter, while in summer it plays the role of an energy sink for the building. The high-temperature circuit consists of a gas-fired CHP, which provides electricity, mainly for the heat pump. Supported by two condensing boilers, which act as a backup, the CHP also generates high-temperature heat for the direct use in the labs or for additional heating, if required. A chiller provides low-temperature cooling for the labs and it is also a backup cooling for the server rooms. Figure 2 shows a schematic illustration of the energy concept of the building.

The control system, called MetaSys, has been implemented in a Building Management System (BMS) by Johnson Controls Inc. The BMS integrates the field, automation and management layers of the control structure, thus it embodies the base automation system of the building. The building has later been upgraded with an extensive monitoring, control and interface system, which enables a high monitoring degree and the ability to get a detailed insight of the interrelations and interactions of all systems for the research work. These specific features provide the functionality of a scientific test bed.
The building has different kinds of varying demands. Server and LAN room, offices, conference rooms, common areas and CIP-Pools have fluctuating demand for cooling, heating and ventilation depending on the internal heat gain and the outside weather conditions over the whole year. In this study, a set of three conference rooms next to each other in the ground floor has been selected a TSoS for implementation of decentralized PCAO. There are two reasons behind this decision. Firstly, the selected system meets the criteria of a system of constituent subsystems. Secondly, since this set of rooms has been so equipped to be used as test bed, it satisfies the conditions of observability and controllability. A Concrete Core Activation system (CCA) meets the base load for heating and cooling while the combination of an Air Cooler (AC) and a Volume flow Controller (VFC) covers the peak load. The VFC regulates the volume flow of the air flow coming from the central air handling unit. The AC provides additional heating or cooling, and its operation stops if any of room windows (WS) gets open. Figure 3 presents a schematic overview of the conference room system with all controllable constituent systems and sensors.
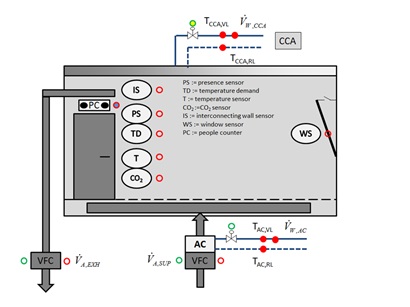
The user interface contains a button (PS) for presence and a dial for temperature demand (TD) “+” and “–“and a sensor (T) that measures the local temperature as “room temperature”. The set point can be adjusted by user from 23°C by 3 °C in each direction via this panel. Moreover, a CO2 sensor (CO2) measures the CO2 concentration to ensure it remains in the acceptable range. Folding walls enable these rooms to be connected, if required, thus the size and capacity is flexible. The state of the folding wall is detected by a pin (IS). To accurately estimate the internal heat gain a person counter is currently being installed in each of these three rooms (PC).
Cost Function:
The used cost function considers non-renewable energy consumption (excessive renewable energy consumption) and the level of comfort:
where 0 < t < 1 regulates the importance of one term with respect to the other. The is derived by reading the actual real-time non renewable energy consumption (NREC) [kJ] required in total. The
is the user comfort index discussed before defined as a temperature penalizing paraboloid with a user defined temperature satisfying area. The lower and upper bounds for the temperatures were chosen according to ANSI/ASHRAE standards for indoor air quality and thermal comfort between [21°C, 25°C] and [400ppm, 1100ppm] for indoor temperature and CO2 concentration levels respectively.
Finally in order to establish an even more elaborate and detailed design, a filtering scheme was adopted so as to take into account comfort score values only during working days and periods [8:00 – 17:00].
Real-Life Results:
Experiments took place during August 2016 and November 2016. The experimental goal was to define energy and comfort level efficient control strategy for heating and cooling purposes respectively. Note that the comparison performance benchmark (Base Case Scenario – BCS) used for comparison purposes is an elaborate, commercial control practice adopted in the real-life building (MetaSys by Johnson Controls Inc.). In parallel, at the same time, to decentralized PCAO application, similar rooms employing the BCS control strategy were used in order to establish a fair post-evaluation process. It has to be underlined that the indoor temperature and CO2 levels, during working period, both for decentralized PCAO and BCS, were pretty much always between the acceptable defined bounds (see above) except very few 15min intervals when both BCS and PCAO decentralized approaches presented CO2 concentrations slightly over the upper bound. In other words, the internal comfort levels were pretty much the same, achieving acceptable comfort levels in both cases.
It could be observed, that L4G was capable to reduce NREC continuously. The periods, where this reverses (when the distance decreases a bit) prove the different control behaviour. It was also visible, that the improvement in summer period was not as high as in autumn period, similarly the absolute values.
The reasons for that are multiple, but mainly it is a higher sensitivity of modern buildings against cooling loads than against heat loads. In summer, the cooling loads of the weather and the internal loads by occupants, devices and light compound the total cooling load, in autumn, they compensate each other. The better the insulation of the building is the greater becomes the effects influence.
At the end, L4GPCAO improved the NREC in the building use case about 27,5 % in average, thus a quarter of fossil energy was consumed during L4GPCAO operations without a reduction of thermal comfort.